Experiments with superheavy elements
The SHE (SuperHeavy Elements) section
Above a certain size, atomic nuclei become increasingly unstable. The heaviest element occurring in nature is uranium with the atomic number 92. Even heavier elements can be produced artificially – partly in nuclear reactors, partly with accelerators. At the Helmholtz Institute Mainz, such superheavy elements are the focus of the SHE (SuperHeavy Elements) section. The analysis of these elements enables both the models of nuclear physics and the theories of chemistry to be tested and improved.
The HIM researchers produce the superheavy nuclei at accelerator facilities such as those of GSI Helmholtzzentrum für Schwerionenforschung in Darmstadt. There, an accelerator fires medium-heavy ions such as zinc or calcium onto a target, consisting for example of lead. Some of the projectile nuclei hit a target nucleus in such a way that they fuse to form a superheavy element. This can be separated from other particles with the SHIP velocity filter or the TASCA gas-filled separator. To study the short-lived nuclei, they are either implanted directly into a suitable detector that measures their radioactive decay, or they must be decelerated for further study. The “brake” is for instance a cell filled with helium or argon. The scientists can then investigate the physical and chemical properties of the nuclei: the particles can be stored in traps, for example, illuminated with lasers or examined regarding their chemical binding behavior to specific substances.
The physical properties are mainly related to the question of why certain isotopes are more stable than others. The experts suspect that some variants of the (still hypothetical) elements 120 and 126 could be unusually stable – some even with lifetimes of years. In the landscape of the superheavy atomic nuclei, these isotopes would stand out like an island from an ocean of unstable ones – the experts speak of the “island of stability”.
The experiments reveal what happens when, for example, you pack more and more neutrons into a nucleus, thereby changing the balance between the attractive strong interaction and the repulsive electromagnetic force of the protons: a few more neutrons can greatly extend the lifetime – suggesting that one approaches the island of stability. Although there are theoretical models today that qualitatively describe this phenomenon, there is still a need for explanation at the fundamental level – all the way to the distant vision of being able to describe atomic nuclei with the fundamental laws of the strong interaction. The analysis of the superheavy elements helps to validate today’s models and develop future, more refined theories.
The experts of the SHE section study the nuclei using different methods, for example laser spectroscopy. For instance, to analyze nobelium (element 102), they decelerate the nuclei in an argon-filled chamber after they have been produced. The nobelium can then be electronically excited with laser light and precisely measured – whereby the behavior of the electrons yields detailed information about the conditions in the atomic nucleus. Subsequently, a detector records the radioactive decay of the unstable nucleus, whose half-life can range from a few seconds to several minutes, depending on the isotope. The results provide information on the change in size of nobelium isotopes with increasing neutron number. They also show that the nobelium isotope with 152 neutrons features maximum deformation. The measurements furthermore confirm theoretical predictions of a central depression in the proton density distribution of these very heavy nuclei..
In other experiments, the decelerated ions are caught in particle traps. These Penning traps can store single ions in strong electromagnetic fields. In the trap, the superheavy ions perform a circular motion in the magnetic field. From the frequency of the motion, the mass can be inferred, from which the binding energy of the atomic nucleus can in turn be determined using Einstein’s famous E = mc2 relation. By comparing isotopes with different neutron numbers, the researchers can find out how the binding energy changes as a function of neutron and proton numbers. As a result, the strength of the closed neutron shell of the nobelium isotope with 152 neutrons is now precisely known. The closed neutron shell leads to a relatively high binding energy and a comparatively high stability.
Among other things, these studies make it possible to assess whether superheavy elements can form when two neutron stars merge – after all, such mergers provide extremely many neutrons for nucleosynthesis. Current observations, combined with the detection of gravitational waves caused by a collision of two neutron stars, show at least that heavy elements such as gold and platinum are produced in the collision, a scenario that was expected for a long time but only recently experimentally confirmed.
Another focus of the SHE section is the investigation of the chemical properties of superheavy elements. In heavy elements, the large proton number significantly influences the chemistry of the element. The reason is that the electrons on the orbitals of the atomic shell that are near the nucleus “feel” the very strong positive charge of the proton-rich nucleus and are accelerated to up to 80 percent of the speed of light, inducing a noticeable relativistic mass increase. The electrons literally gain weight and reduce their radii of motion around the atomic nucleus. This in turn affects the shape and size of the electron orbitals, which determine the chemical properties. Among other things, such relativistic effects cause gold to have its typical color and mercury to be liquid instead of solid at room temperature.
In the superheavy elements, these relativistic effects are even more pronounced due to the very high nuclear charge – which is why it is worth analyzing them in detail. For example, the HIM researchers are investigating the chemical properties of flerovium, element 114. According to the periodic table, it should have similar chemical properties to lead as it has the same electron configuration. However, relativistic effects could cause element 114 to behave like a noble gas rather than a heavy metal. To clarify the question, an experiment is required. The challenge is that only a few flerovium atoms per day can be generated in accelerators – the experts have to do chemistry with a single atom. In addition, the isotopes live only about a second, meaning that the experiment needs to be performed just as quickly.
To this end, the scientists decelerate the flerovium atom and direct it into a thin, 30 centimeter long channel. The gold-plated channel is warm at the front and cold at the back. The idea is that if element 114 behaves like lead, it should stick to the inner wall of the channel in the front area and form a metal bond with the gold. In contrast, if it resembles a noble gas, it should not bind to the gold at the beginning of the chamber but fly to the minus 170 degree cold end of the channel, where it should be adsorbed by van der Waals forces.
Alpha detectors are placed along the entire channel, registering the decay of the flerovium nucleus and revealing whether it stuck in the front or arrived at the back. So far, different research teams have come to different conclusions: a Swiss-led collaboration working in Dubna, Russia, identified element 114 as a noble gas – a behavior that the Mainz researchers were unable to confirm with their first measurements. Since then, other more detailed experiments have been performed, which are now being evaluated.
In addition, the HIM researchers even synthesize molecules with superheavy elements, such as compounds with carbon monoxide, so-called carbonyl complexes. Recently, for example, the experts succeeded in synthesizing a seaborgium carbonyl complex, in which element 106 combines with six carbon monoxide molecules. Subsequently, they examined how the short-lived molecule interacts with a quartz surface. The analysis of the corresponding binding strengths allows conclusions to be drawn about the chemical reactivity of the complexes and provides insight into the chemical properties of the heaviest elements. The experiments thus help to validate the current models of theoretical chemistry.
The HIM activities conducted within the EU project “nuClock”, in which the section participates as an associated partner, are also a success. The aim here is to develop the foundations for a “nuclear” clock, which could potentially be more accurate than today’s atomic clocks. The isotope thorium-229 has a long-lived excited state at an unusually low energy of only a few electronvolts – which is the same order of magnitude as in electronic transitions in the atomic shell. This led to the idea that, instead of the usual atomic shell, the thorium-229 nucleus could be used to realize the time standard. The advantage is that the atomic shell shields the nucleus very well against external disturbances, which is why a nuclear clock could “tick” significantly more accurately than an atomic clock.
Some time ago, the nuClock team was able to directly detect this low-energy state in thorium – a result that made it into the top ten list of the British Institute of Physics (IOP) in 2016. The HIM had contributed to the production of the thorium-229 source for these experiments. It is based on thin, homogeneous layers of uranium-233. The uranium decays by alpha decay into thorium-229, thereby generating excited nuclei, among others. These recoil from the layer during the uranium decay and are available for analysis. Further work now focuses on characterizing the properties of the excited thorium state.
Prof. Dr. Christoph E. Düllmann
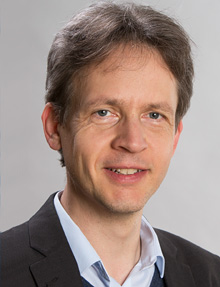
Helmholtz Institute Mainz
Staudingerweg 18
D-55128 Mainz
Room 02-119
Phone: +49 6131 39-25852
E-Mail: duellmann(at)uni-mainz.de
Mainz, Department of Chemistry - TRIGA Site
Darmstadt, GSI - SHE Chemistry
Room Room GSI SB3.3.173
Phone: +49 6159 71-2462
Prof. Dr. Michael Block
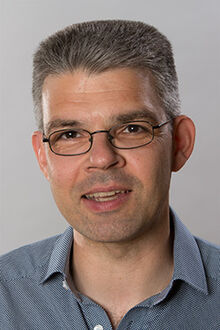
Helmholtz Institute Mainz
Staudingerweg 18
D-55128 Mainz
Room: 02-121
Phone: +49 6131 39-29683
E-Mail: block(at)uni-mainz.de
Darmstadt, GSI
Room: GSI SB3.3.164A
Phone: +49 6159 71-2845