Calculations on the lattice
The THFL (Theory Floor) section
Quantum chromodynamics (QCD) is considered a highly powerful theory for describing the strong interaction, that is, the force acting between the quarks. However, it is still a big challenge to calculate specific quantitative values from the fundamental equations of this theory, such as the masses and structural properties of mesons and baryons. The THFL (Theory Floor) section of the Helmholtz Institute Mainz develops and uses sophisticated computer simulations for this purpose.
Accelerator experiments—such as those conducted by the BESIII collaboration in Beijing, which also involves the HIM—produce and analyze exotic particles or particle states, especially mesons (particles consisting of two quarks) and baryons (systems composed of three quarks). However, whether the properties of these experimentally well-studied particles can be calculated using today’s known formulas and physical laws is an open question: Is QCD suitable for making quantitative predictions in this area? Is it understood in such depth that it can precisely explain the rich spectrum of observed particles?
To answer these questions, one needs to apply quantum chromodynamics in the low-energy range. This is extremely difficult, however, because unlike in the high-energy regime, no approximation methods can be used at low energies. The experts therefore rely on complex numerical simulations, which run exclusively on mainframe computers.
For this purpose, the HIM theorists use the method of lattice QCD, in which the continuous space-time is replaced by a discrete lattice of points. The fields of quarks and gluons then exist only at the lattice points and their connections. In this way, it becomes possible to solve the fundamental equations of QCD by computer.
Nevertheless, the calculations are so extensive that they can take months even on a supercomputer: To be able to extrapolate from the discrete lattice to the real continuum, the researchers have to cover certain parameter ranges and repeat the simulations several times, each time using different values for the lattice constant and the quark masses. In a way, the methodology resembles the computer models of climatologists: Here too, the scientists work with lattice models that are run in different variants, thereby yielding an ensemble of results.
For their calculations, the HIM theorists can resort to an impressive hardware, in particular the institute’s own CLOVER computer cluster. Its 5,120 processor cores offer a processing power of 120 teraflops, with a usable main memory of 10 terabytes. Currently, the capacity is being doubled to around 10,000 processors.
The scientists also have access to the MOGON II computer in Mainz, in which the HIM is involved. MOGON II is currently the fastest high-performance computer at a German university and ranks among the 100 most powerful supercomputers in the world. Around 49,000 processor cores provide a computing power of two petaflops, and the storage features a capacity of 7.5 petabytes.
With their computer models, the HIM theorists determine the energy levels and decay rates of particle states. Among other things, they are investigating the binding states of entities that consist of more than three quarks—for example, so-called hexaquarks or dibaryons. These are made up of a total of six quarks and form the simplest system one could use to theoretically understand nuclear matter based on QCD. Researchers already seem to have found first experimental indications for the existence of such hexaquarks.
Studying the structure of hadrons is equally important: How are the quarks and gluons distributed inside them, how are the electric and magnetic charge, for example, distributed inside the proton? To find out, the HIM theorists use lattice QCD to calculate so-called form factors. Among other things, they compare their results with the values measured at the MAMI accelerator in Mainz.
In addition, the physicists of the THFL section investigate how matter behaves under extreme conditions. Thus, in the early cosmos, an extremely dense and hot state of matter may have predominated—the quark–gluon plasma. By means of lattice QCD, the scientists can calculate how high the electrical conductivity in this plasma is and at what rates certain particles are created inside it. The HIM theorists then compare their results with experimental data, obtained for instance at the US accelerator RHIC or the LHC at CERN.
Another quantity the theorists are targeting using lattice QCD is the anomalous magnetic moment of the muon. In accelerator experiments, the muon—a kind of heavy brother of the electron—seems to show a remarkable deviation from the predictions of the Standard Model of particle physics. Should this discrepancy also be observed in future, far more precise experiments, a crack could open up in the theory—an exciting hint at physics beyond the Standard Model. The HIM theorists strive to calculate the so-called hadronic corrections as accurately as possible—an important prerequisite for the precise comparison of the measured values with the theoretical predictions.
Finally, the THFL section also deals with the physics of light nuclei. Here, QCD can no longer be applied. Instead, the experts use sophisticated analytical methods to develop and refine so-called effective theories, which arise from certain limiting cases of quantum chromodynamics. These effective theories are based on specific symmetry principles that can be used to classify the interactions between the nuclear particles. The aim of the work is to predict the binding energies of light atomic nuclei as accurately as possible.
Prof. Dr. Hartmut Wittig
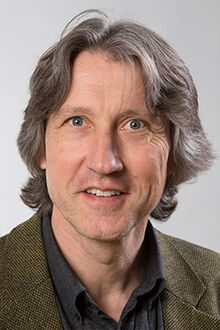
Helmholtz Institute Mainz
Staudingerweg 18
D-55128 Mainz
Room 2-100
Phone: +49 6131 39-26808
E-Mail: wittig(at)kph.uni-mainz.de