Innovative concepts for better accelerators
The ACID (Accelerator Design and Integrated Detectors) section
Accelerators and detectors are among the key tools of particle, hadron and nuclear physics. Without them, it would be impossible to penetrate ever deeper into the microcosm, for example to bring to light novel details about strong interaction. In the design of accelerators and detectors, the experts push the boundaries of what’s technologically feasible. Instead of being able to rely on standard components, they often have to plan, develop and realize completely new components.
At the Helmholtz Institute Mainz, the ACID (Accelerator Design and Integrated Detectors) section is working on such innovative accelerator technologies. In close cooperation with other sections of the HIM, the ACID section devises concepts that will enable significantly better and more precise experiments. The focus is on two projects: One team is developing a novel superconducting accelerator that will enable superheavy chemical elements to be produced much more effectively than before. The second half of the section is working on very high-power electron beams which are superimposed with high energy ions in storage rings, in particular at FAIR. Such a scheme, called electron cooling, increases the productivity of experiments at the ion rings considerably. These developments have recently created a surprising spin-off, using high power electron beams for an innovative form of radiation therapy.
Ion accelerator in continuous operation - the HELIAC
In order to produce superheavy chemical elements, accelerators fire medium-heavy ions of calcium, titanium or chromium onto a target. On impact, some of the accelerated ions can fuse with atomic nuclei of the target into “superheavy” elements. So far, the accelerators of GSI Helmholtzzentrum für Schwerionenforschung in Darmstadt provide pulsed beams, i.e. the facilities fire series of bursts. The ACID section is working on new accelerator technology that provides a continuous, i.e. uninterrupted ion beam. This should make the future production of superheavy elements much more efficient than before.
One difficulty in producing superheavy elements in the laboratory is that the reactions between the fast ions and the atomic nuclei of the target happen only very rarely. To nevertheless achieve a measurable yield, the physicists must accelerate as many ions as possible and deliver them to the target with the necessary reaction condition. With the accelerator technology used so far, the ions are bundled into short pulses. This has a disadvantage for the target material, as the pulses put a heavy load on it in a short time, in extreme cases even threatening to destroy it.
More favorable would be a load in which the ions do not pounce on the target in violent bursts but hit it steadily and continuously in the so-called continuous-wave mode. In a way, the situation is similar to a glass of water that you want to fill to the brim: if you pour the water with too much momentum, you will probably spill part of it. A more promising approach is to pour the water into the glass in a smooth, not too strong jet.
However, such a continuous-wave mode is difficult to achieve with conventional accelerator technologies. That’s why the HIM physicists are working on a new approach: they are developing radio-frequency cavities (accelerating tubes) based on superconductivity. In superconducting materials, electric current can flow without loss and without resistance, provided the conductors are cooled with liquid helium to temperatures near absolute zero at minus 273 degrees Celsius.
On the one hand, such superconducting cavities enable very high accelerating gradients, i.e. the ions are accelerated very strongly over short distances. On the other hand, the cavities exhibit little loss, so they use less energy than conventional ones. These two advantages more than outweigh the disadvantage of the elaborate helium cooling. It was decided that this innovative new accelerator deserves a dedicated name – it is called the HELmholtz Linear ACellerator – or HELIAC for short.
Although there are already superconducting accelerators in the world, such as the electron accelerator of the European XFEL X-ray laser in Hamburg, the cavities for the planned continuous-wave accelerator for heavy ions in Darmstadt must have a much more complex shape than those in existing facilities: in contrast to the relatively simply formed European XFEL cavities, the heavy-ion cavities from Mainz, which are made of the metal niobium, need a complex inner structure consisting of several cross-shaped arrangements with integrated drift tubes.
In addition, the cavities are not all identical. Each of them is manufactured individually. The reason for the differences is that the facility will not accelerate lightweight electrons, all of which move at almost the speed of light, but much heavier ions. At the beginning of the accelerator, these are very slow and gain momentum only gradually. As a consequence, each cavity must be adapted individually to a specific speed range and thus differ from the others. Twelve cavities in total are planned for HELIAC. It is to have an overall length of about 15 meters and to accelerate the ions up to almost ten percent of the speed of light. Since experiments are planned at different energies, the accelerator must feature a high degree of flexibility.
The development challenges are huge: the HIM experts have to manufacture the complex mechanical structure with high accuracy – the positioning of the elements should be accurate to a few micrometers. In addition, how superconductivity behaves in such complex structures under high radio-frequency power during beam operation still needs to be investigated.
Together with a specialized company, the HIM researchers built the first cavity of the accelerator. The subsequent testing on a test bench was successful: the targeted accelerating gradients were not only achieved but significantly exceeded.
Next, ACID-physicists have equipped a complete cryomodule of HELIAC with a total of three accelerating cavities which were complemented by very strong solenoid lenses. In such a cryomodule the cavities are cooled down towards -269 degree Celsius, just 4 degrees above absolute zero. After assembly in the HIM clean room the whole cryomodule was transported towards GSI, where first successful operation with an ion beam was achieved just before X-mas 2023. Meanwhile the first full cryomodule of HELIAC has fulfilled the specifications regarding accelerating capabilities.
Presently scientists of ACID are enthousiastically working to assemble the full HELIAC which is to be operated at GSI before the end of the decade. With HELIAC, the researchers of the SHE (SuperHeavy Elements) section will produce and investigate superheavy chemical elements much more effectively than before.
High power beams for electron cooling and medical applications
Electron cooling is mandatory if one wants an optimum exploitation of experiments installed at an ion storage ring. Several such rings are planned at GSI-FAIR. ACID scientists are prepared to provide technical solutions for electron coolers and improving the state of the art for the storage rings at FAIR.
For instance, the HESR high-energy storage ring will be one of the core elements of the FAIR accelerator complex under construction in Darmstadt: it will accelerate and store ions, in particular antiprotons. The fast antiparticles enable novel, highly promising experiments with the planned PANDA detector, which will be inserted into the beam path of the HESR storage ring.
In the PANDA experiments, at each revolution around the HESR ring the stored antiproton beam hits a target consisting of a beam of microscopic frozen hydrogen pellets. The ensuing collisions create new, exotic particle states, which PANDA will measure precisely. The beam traverses the target about one million times per second. Each time, only a few antiprotons in the beam collide with the protons in the target. Most ions only cross the target, remain in the ring and continue on their laps.
The problem is that, in these frequent crossings, the antiprotons are deflected a bit by the target—some to the right or left, others up or down. As a consequence, irregular motion is added by the target to the otherwise very well defined orbit of the ions. Since irregular motion corresponds to temperature, the ion beam heats up - equivalent to temperatures of several millions of degrees. In consequence, due to the chaotic motion associated with the extreme temperature, the laboriously produced antiproton beam will be lost after a very short time. This can be prevented if an intensive beam of electrons is superimposed on the antiproton (or ion) beam which travels at the same velocity. Then, the electrostatic forces between the two beams lead to a continuous transfer of irregular motion (“the heat”) from the stored ions to the electrons - which have a temperature of only about 1000 degrees Celsius - and are continuously replaced by new “cold” electrons. Therefore, the ion beam gets continuously cooled and can be sustained for the experiment in spite of the heating by the target.
The concept poses several challenges. The electron beam must have a high intensity exceeding 1 Ampere of current. To achieve the same speed as the antiprotons, the electrons need to have a kinetic energy of eight Mega-electronvolts, corresponding to a power of more than eight Megawatts. The generation of such a power at a voltage of eight megavolts will be outside the technological possibilities for the foreseeable future and would also be questionable from an ecological point of view due to the enormous energy requirement. Therefore, more than 99 percent of the kinetic energy is to be recovered by decelerating the electrons to an energy of a few kilovolts only after the interaction with the ion beam has happened. This energy recovery requires extremely precise control of the electron beam—after all, the power levels are so great that a misdirected beam could cause damage to the accelerator. The ACID team has meanwhile demonstrated the acceleration of 1Ampere electron beam at 30000 Volts in a small test set-up. The non-invasive detection of the position and the size of the beam was demonstrated by observing the fluorescence radiation caused by the extremely weak interaction of this beam particles with the residual gas. Therefore, the beam can be properly controlled, and less than one in a million electrons is lost while travelling through the apparatus.
Focusing of the electron beam is achieved using special magnetic lenses housed in the electrostatic acceleration section. Supplying electric power to these lenses is a tricky undertaking, as supply via cables used in conventional high voltage electronics does not work at the extreme voltages needed here. This is why the HIM scientists have found an innovative solution: the energy for operating the magnetic lenses and the electron source comes from special turbine generators driven by compressed gas. The gas can be transported in insulated pipes, which are not affected by the high voltage. The turbine runs at a speed of about 30000 turns per minute and delivers 3kW of power which is more than enough to power the focusing lenses.
Physicists are currently building a module that runs at a high voltage of 600,000 volts. ACID wants to demonstrate the scalability of these modules by staging them with the long-term goal to reach HESR-energies or to be used at any other ion ring with lower energy. The experimental hall of the HIM is used for the construction and testing of these modules.
Incidentally, the University of Mainz and GSI Helmholtzzentrum für Schwerionenforschung also use another section of this large experimental area for mounting superconducting accelerator modules, for example for MESA. This new electron accelerator for particle and hadron physics is currently being developed as part of the Excellence Initiative.
Handling high power beams for electron cooling unexpectedly created perspectives in a completely different field, namely cancer therapy. In a collaboration with TU-Munich and Forschungszentrum Jülich, ACID researchers have developed the so-called Line-focus-X-ray tube, a device which promises efficient radiation therapy with significantly reduced side effects compared to existing methods. This is based on the production of X-rays which are distributed in lines of a very small lateral dimension. These X-rays are generated by an electron beam which is focused on a thin line on the anode of the X-ray tube. The electron source and the beam focusing system were designed by ACID-scientists at assembled in the HIM-cleanroom. It is known to be installed at the medical research center at TU-Munich where first tests on animals have been performed in the summer of 2024. Several developments, in particular increase of the beam power and the accelerating high voltage are under investigation. If successful, this can lead to a clical device in the medium future.
Prof. Dr. Kurt Aulenbacher
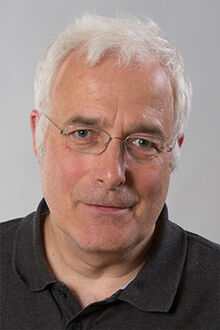
Helmholtz Institute Mainz
Staudingerweg 18
D-55128 Mainz
Room 02-105
Phone: +49 6131 39-25804
E-Mail: aulenbac(at)kph.uni-mainz.de
Prof. Dr. Winfried Barth
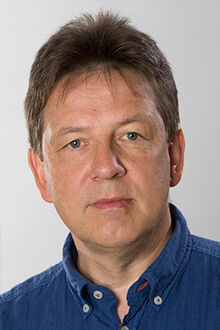
Helmholtz Institute Mainz
Staudingerweg 18
D-55128 Mainz
Room 01-105
Phone: +49 6131 39-29217
E-Mail: w.barth(at)gsi.de